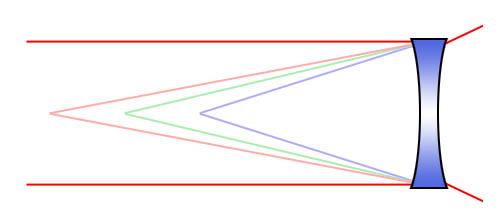
Optical Designs
The following section describes in detail how various optical components work to form an image in a telescope, and how they modify or correct the aberrations present. This is intended to give more information to those who want to know exactly how all the components in their telescopes--from mirrors to focal reducers--function. It will also help explain why there are so many different designs available and what are the advantages and disadvantages of different designs.
Mirrors
The main purpose of a telescope is to gather light. Reflecting telescopes do this using mirrors. There are a variety of reflecting optical designs in existence, and each uses a different combination of mirror types to produce different results in terms of focal length, focal ratio, and aberrations present.
Concave Mirrors
The primary mirror in a reflecting telescope is a concave mirror, designed to focus incoming light to a point. The converging light cone from this mirror can then be intercepted by another optical component to modify the beam, or the focused light can be sent to an eyepiece or camera. The greater the curvature of the mirror, the shorter the distance to focus, the focal length. Deeper curves are harder to manufacture and have inherently more aberrations. They do, however, allow a shorter overall instrument and faster (smaller) focal ratio.
Convex Mirrors
Convex mirrors disperse light rather than focus it. They are used in conjunction with a primary concave mirror. The effect of placing a convex mirror into a converging light path is to extend the distance to the focal plane. This increases the effective focal length and focal ratio. This is howCassegrain telescopes create a long focal length in a short tube. The greater the curvature of a convex mirror, the more it disperses light and the longer the resulting focal length will be.
Above: How a Cassegrain telescope creates a long effective focal length in a short tube
Aspheric Mirrors
Mirrors can be ground into various shapes. The simplest and easiest-to-make shape is spherical. A single spherical mirror cannot, however, focus light to a single point. The only shape that can achieve this is a parabola. This is why satellite dishes and the audio dishes along the sidelines of NFL games are parabolic. Newtonian telescopes are a single-mirror design (if the flat diagonal mirror which has no optical effect is ignored), and therefore must use a parabolic mirror to focus light.
Above: A spherical mirror does not focus all incoming light to the same point
Above: A parabolic mirror focuses all incoming light to a single point
Cassegrain telescopes must also use aspheric mirrors to create sharp images. Depending on the design, the mirrors may be parabolic, hyperbolic, ellipsoidal, or even spherical (as long as at least one is aspheric). See the box below for details on the different shapes. Commercial Schmidt-Cassegrain telescopes (SCTs) normally use two spherical mirrors and the resulting aberrations are corrected with a lens--see the next section for details on lenses.
Understanding Mirror Shapes A variety of shapes are used in manufacturing various telescopes. Below are the different possible shapes for mirrors and the telescopes that use certain combinations of mirrors and the reasons for those mirror choices in a design. Spheroidal -- The simplest curved mirror shape is that of a sphere. Simply grinding two pieces of glass together will yield a spheroidal shape. Paraboloidal -- A parabola is a slightly more complex shape than a sphere, but is not too much more difficult to make. This is the simplest of the aspheric (non-spherical) shapes. Hyperboloidal -- A hyperbola has a more complex shape than a parabola and is thus very difficult to manufacture. Hyperbolas are larger curves than parabolas (see below). Ellipsoidal -- Ellipses are smaller curves than parabolas but are still difficult to manufacture. The image below shows the mathematical shapes behind each mirror design. Above: The black line represents a flat mirror. Only two curved shapes have exact specifications: the sphere and the parabola. The orange line represents a sphere, which is defined by only one mathematical equation. The green line represents a parabola, also defined by a specific equation. Ellipses and hyperbolas, on the other hand, have infinite variety. The blue line represents a specific hyperbola, but any curve in the blue section of the graph would be a hyperbola. Likewise the yellow curve represents a prolate ellipse (in which the foci are parallel to the optical axis--in other words, the ellipse's long axis runs along the optical axis), but all the curves within the yellow area would be prolate ellipses. The same is true for the oblate ellipse family, which inhabits the red part of the graph. Oblate ellipses have their long axes perpendicular to the optical axis. |
Lenses
Refracting telescopes use lenses rather than mirrors to focus light to a point. This complicates the design since multiple lenses must be used and only certain combinations of glass types will produce a functioning telescope objective. Binoculars use the same principles since a binocular is essentially two small refractors hooked together. Lenses are also used to correct aberrations in some reflecting telescopes and are the basis for the accessories outlined in the next section.
Positive Lenses
A positive lens causes incoming light to converge, analogous to a concave mirror. The difficulty in using a lens instead of a mirror comes from the fact that lenses refract light rather than reflect it. Reflected light behaves independent of wavelength; all the colors focus to the same point. This is not true of refracted light. A simple lens focuses different wavelengths of light to different distances from the lens. Essentially the lens has a different focal length in each color. For this reason, a single positive lens is not sufficient to make a functional telescope.
Negative Lenses
A negative lens causes incoming light to diverge, similar to how a convex mirror operates. A negative lens is used in combination with a positive lens to correct the color aberrations of a single lens. If the right types of glass are chosen, the color aberration of the negative lens will cancel that of the positive lens. The result is an overall positive lens, but with less color aberration. It turns out that with two pieces of glass, the aberrations can only be exactly cancelled for two wavelengths. But if the wavelengths are chosen right, the remaining aberration is very small and a well-corrected telescope is the result. A lens of this type is called achromatic. Using more than two pieces of glass allows for even better correction, resulting in an apochromatic lens.
Glass Types
In a typical doublet objective (where two pieces of glass are used, as described above), the two components must have carefully selected properties to allow them to work as an achromatic lens. There are hundreds of glass types available, but they can be grouped into two families: crowns and flints. One of the two main properties of a glass type is its dispersion. Dispersion describes how much a glass spreads out the different colors of light. The greater the dispersion, the more the light is spread out. Glasses with higher dispersions are called crowns, while those with lower dispersions are called flints. Combining a high-dispersion crown with a low-dispersion flint allows an achromatic lens to be made. Certain combinations work better than others, but in general the lower the dispersion of the flint, the better the overall color correction of the lens. Hence the use of extra-low dispersion (ED) glass in high-quality refractors.
Corrector Lenses
Some reflecting telescopes use a correcting lens to eliminate residual aberrations. Such a telescope is called catadioptric. The most common examples are the Schmidt-Cassegrain and Maksutov-Cassegrain telescopes. These telescopes use a corrector lens, placed over the fullaperture of the telescope, to eliminate the aberrations present from using spherical mirrors in the reflector design. (Some catadioptric telescopes use an aspheric mirror to eliminate other aberrations, but the full-aperture corrector is still required.) The corrector lens in a Maksutov-Cassegrain is spherical but thick, while the Schmidt-Cassegrain uses a thin but aspheric lens. Other telescopes incorporate sub-aperture corrector lenses somewhere in the light path to correct aberrations. Sub-aperture correctors are often seen in Ritchey-Chrétien telescopes to eliminate the inherent astigmatism in the design.
Accessories
A variety of accessories are used to enhance telescope optics. Barlows are used to increase magnification, while focal reducers do the opposite by reducing magnification. Other accessories correct aberrations. All use the same design principles as telescopes themselves.
Barlow Lenses
Above: How a Barlow lens increases focal length
A Barlow lens is essentially just a negative lens. As was seen above, the effect of a negative lens is to disperse light. By dispersing the converging beam from a telescope, a Barlow increases the distance to the focus point, thereby increasing the effective focal length. A standard 2x Barlow has the effect of doubling the focal length of a telescope. A Barlow normally consists of a doublet lens, used to correct any inherent aberrations. The combination of glass is done just as in a telescope objective, only the resulting lens is overall negative rather than positive. Some Barlows incorporate a three-element design, and more advanced designs even use four elements to reduce as many aberrations as possible, allowing for very high-powered viewing or imaging without degrading the image quality.
Focal Reducers
Above: How a focal reducer decreases focal length
A focal reducer works opposite to the way a Barlow functions. It is a positive lens and has the effect of causing the incoming beam of light to converge faster, shortening the focal length. This also decreases the focal ratio of the instrument. The result is a wider field of view and faster photographic speed. A focal reducer is essentially a positive lens as in a refractor (although three to four elements are normally used for the best correction). The shorter the focal length of the focal reducer, the greater the reduction factor when placed into the optical path of a telescope. In addition to the focal length of the reducer being important, the distance from the reducer lens to the focal plane is critical to determining the reduction factor. Placing the focal plane closer to the reducer results in a longer overall focal length, while moving the focal plane away from the reducer decreases overall focal length. As an example, a standard 0.63x focal reducer has a focal length of about 250mm and reduces an f/10 telescope to f/6.3. This is true if the distance from the reducer to the focal plane is about 100mm. If this distance is decreased, the focal ratio will be larger than f/6.3. Increasing this distance will make the telescope faster than f/6.3 (although the image quality suffers).
Field Flatteners
Most telescopes have a curved focal plane, meaning the points of best focus across the field form a curve. Visually this presents little problem. For imaging, however, the curved focal plane will not coincide with the flat plane of the CCD chip in a camera. To correct this, a field flattener can be used. A field flattener is a lens (usually composed of two elements) that is placed in the light path just ahead of the focal plane. The result is to remove or reduce the field curvature and allow the image to focus sharply across the full field of the CCD. Some telescope designs incorporate a field flattener into the telescope to produce a flat field without any accessory optics.
Coma Correctors
Newtonian telescopes inherently have coma, an off-axis aberration that distorts star images. This becomes especially problematic for wide-field imaging, although it can be disturbing visually in large-aperture, fast-focal-ratio telescopes. A coma corrector is a lens placed just ahead of the focal plane which cancels out the telescope's coma, resulting in much sharper stars at the edge of the field. Since the coma corrector is a weak negative lens, the effect is to increase the focal length slightly.